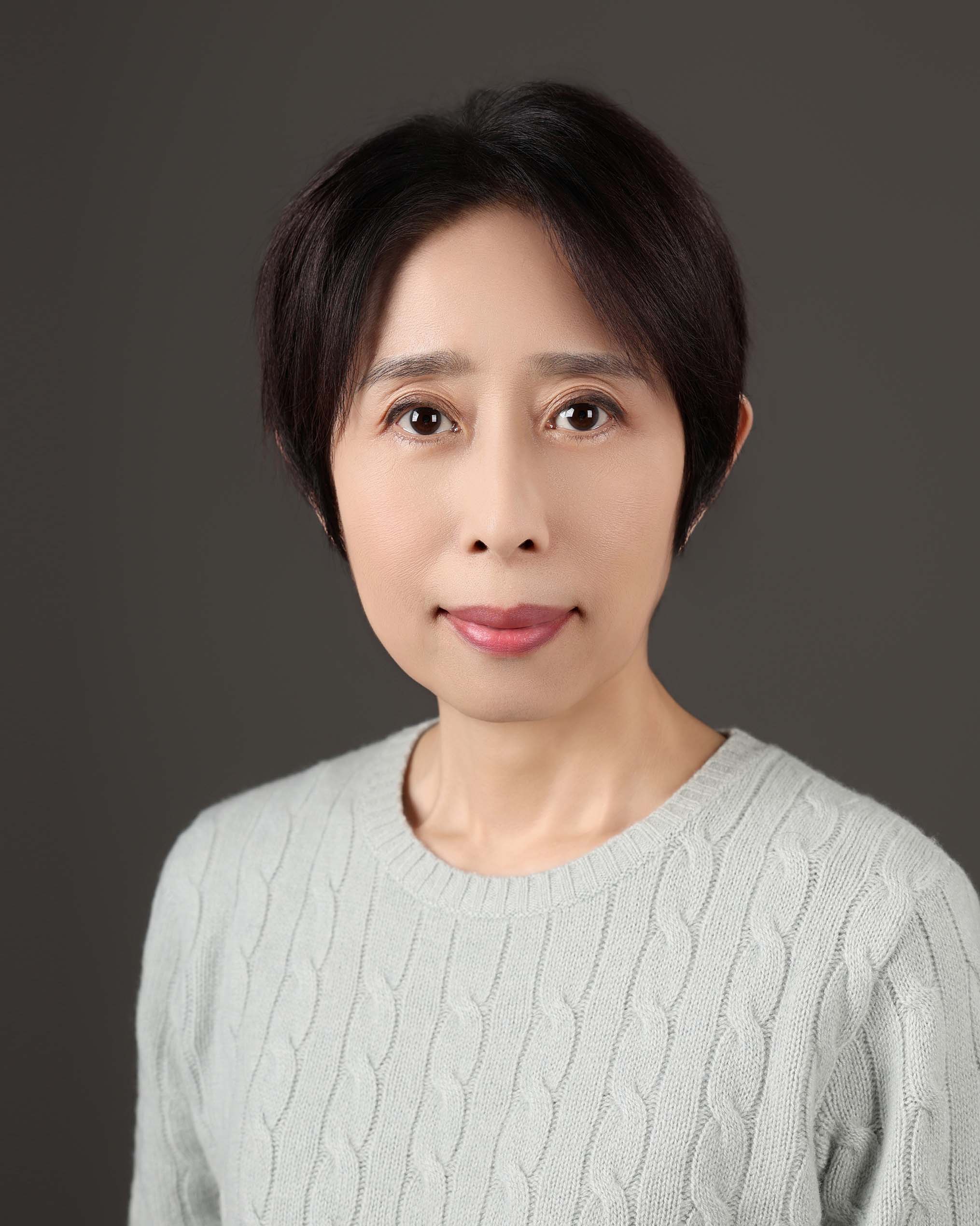
Xuemei Chen, Ph.D.
Plant small RNA and RNA modification group
Professor, College of Life Sciences, Peking University
tel:
E-mail:xuemei.chen@pku.edu.cn
1、Studying the intercellular and long-distance transport of small RNAs, which underlie their roles as signaling molecules in plants’ responses to stresses.
2、Investigating noncanonical RNA caps - detection and identification of noncanonically capped RNAs, uncovering mechanisms of cap deposition and removal, and understanding the biological functions of noncanonical caps.
3、Developing RNA technologies for targeted manipulation and optimization of agronomic traits.
4、Understanding plastid biology, with a focus on plastid gene expression and RNA modifications.
1、Molecular mechanisms of microRNA biogenesis, degradation, and modes of action in plants
We discovered the widespread exist of microRNAs in plants in 2002 (one of the first three laboratories discovering microRNAs in plants), and identified two key genes,DICER-LIKE 1andHEN1, involved in miRNA biosynthesis (Park et al., Current Biology, 2002). In 2005, we found that plant microRNAs carry a 2 '-O-methylation modification on the 3' terminal ribose, which is catalyzed by the methyltransferase HEN1 (Yu et al., Science, 2005; Yang et al., NAR, 2006; Huang et al., Nature, 2009). The homologous protein of HEN1 in animals was later proven by multiple laboratories to be capable of methylating endogenous siRNA and piRNA. Meanwhile, we discovered that the terminal 2 '-O-methylation can protect small RNAs from degradation (Li et al., Current Biology, 2005). It has been demonstrated that the degradation of small RNAs is accompanied by the 3’ truncation and 3’ uridylation, and multiple genes involved in microRNA degradation have been identified, including SMALL RNA DEGRADING NUCLEASE (SDN) and nucleotidyltransferases, the degradation mechanism of plant small RNAs has also been revealed (Ramachandran et al., Science, 2008; Zhao et al., Current Biology, 2012; Zhao et al., Plant Cell, 2013; Ren et al., PNAS, 2014; Tu et al., PLoS Genetics, 2015; Yu et al., PLoS Biology, 2017; Chen et al., Nature Communications, 2018). Subsequently, multiple laboratories in animal research found that microRNAs and microRNA precursors in animals also undergo uridylation. In plants, microRNAs inhibit target gene expression in two manners: mRNA cleavage and translation repression. microRNA-mediated mRNA cleavage has been considered as the main mode of action of plant microRNAs for a long time, our early research found that miR172 represses the protein level of its target gene AP2 but not the mRNA level (Chen, Science, 2004). We further demonstrated that plant microRNAs can indeed mediate translation inhibition of target genes through AMP1 and this process occurs on the rough endoplasmic reticulum (Li et al., Cell, 2013). Subsequent studies showed that plant microRNAs can induce the biosynthesis of a class of endogenous siRNAs (phase siRNAs) on the rough endoplasmic reticulum (Li et al., eLife, 2016).
2. Intercellular movement of microRNAs and their roles in thermomorphogenesis as signaling molecules
microRNAs can act in a non-cell autonomous manner. They not only function in the cells they are produced, but also move between cells, tissues and organisms to regulate the expression of target genes, thereby playing crucial roles in plant growth and development and responses to environmental stresses (Chen et al., Nature Reviews Molecular Cell Biology, 2021). We are currently dedicated to exploring the molecular mechanisms of small RNA movement. Our recent studies showed that disruption of microtubules in Arabidopsis leads to defects in the non-cell autonomous function of mobile microRNAs, revealing the mechanism by which microtubules promote the non-cell autonomous action of microRNAs by inhibiting their cytoplasmic loading onto AGO1 (Fan et al., Developmental Cell, 2022). In addition, we found that microRNAs participate in the regulation of thermomorphogenesis. microRNA156 and its targetSQUAMOSA PROMOTER BINDING PROTEIN-LIKE 9(SPL9) condition auxin sensitivity to enable plant growth plasticity in response to environmental changes (Sang et al., Nature Communications, 2023). Mobile microRNAs may also play important roles in thermomorphogenesis.
3. Noncanonical caps of plant RNAs
RNA modifications are integral processes in gene expression and often play regularoty roles. Among these modifications, the m7G cap modification in eukaryotes affects a series of crucial molecular processes from transcription to translation. In recent years, we discovered a novel non-canonical cap modification at the 5' end of mRNA in plants – the NAD+cap. Our preliminary findings suggest that NAD+capped RNAs undergo splicing, polyadenylation, and recruitment by ribosomes, thereby suggesting their participation in protein translation (Wang et al., PNAS., 2019; Zhang et al., PNAS., 2019). Furthermore, we developed various efficient, sensitive, and specific methods, such as liquid chromatography-mass spectrometry (LC-MS) and high-throughput sequencing, to detect and profile RNAs with several non-canonical caps in bacteria and plants, including NAD+, FAD, and dpCoA (Hu et al., PNAS., 2021; Zhang et al., PNAS., 2021). To better understand the functions of these non-canonically capped RNAs, we identified decapping enzymes capable of specifically removing these non-canonical caps and characterized their decapping activities. Additionally, we constructed the corresponding Arabidopsis mutants to elucidate the biological functions of non-canonically capped RNAs, with a focus on plants’ responses to abiotic and biotic stresses.
Wang XF, Yu DL, Yu JC, Hu H, Hang RL, Amador Z, Chen Q, Chai JJ, Chen XM. (2024) Toll/interleukin-1 receptor (TIR) domain-containing proteins have NAD-RNA decapping activity. Nat. Commun., 15: 2261.
Hang RL, Li H, Liu WJ, Wang RY, Hu H, Chen M, You CJ, Chen XM. (2024) HOT3/eIF5B1 confers Kozak motif-dependent translational control of photosynthesis-associated nuclear genes for chloroplast biogenesis. Nat. Commun., 15: 9878.
Hang RL, Xu Y, Wang XF, Hu H, Flynn N, You CJ, Chen XM. (2023) Arabidopsis HOT3/eIF5B1 constrains rRNA RNAi by facilitating 18S rRNA maturation. PNAS, 120: e2301081120.
Wang XF, Yuan D, Liu YC, Liang YM, He J, Yang XY, Hang RL, Jia H, Mo BX, Tian F, Chen XM, Liu L. (2023) ID1 functions as an autonomous phosphate (Pi) regulator upstream of the miR399-ZmPHO2 signaling module in maize. Plant Cell, 35: 2208-2231.
Sang Q, Fan LS, Liu TX, Qiu YJ, Du J, Mo BX, Chen M, Chen XM. (2023) MicroRNA156 conditions auxin sensitivity to enable growth plasticity in response to environmental changes in Arabidopsis. Nature Comm., 14: 1449.
Xu Y, Zhang Y, Li ZF, Soloria A, Potter S, Chen XM. (2023) The N-terminal extension of Arabidopsis ARGONAUTE 1 is essential for microRNA activities. PLoS Genet., 19: e1010450.
Wang Y, Le BH, Wang JQ, You CJ, Zhao YH, Galli M, Xu Y, Gallavotti A, Eulgem T, Mo BX, Chen XM. (2022) A novel factor that recruits and excludes Pol IV-mediated DNA methylation in a site-specific manner. Sci. Adv., 8: eadc9454.
Fan LS, Gao B, Xu Y, Flynn N, Le B, You CJ, Li SF, Achkar N, Manavella PA, Yang ZB, Chen XM. (2022) Arabidopsis AAR2, a conserved splicing factor in eukaryotes, acts in microRNA biogenesis. PNAS, 119: e2208415119.
Dong HJ, Wang XF, Tan C, Gao L, Cui J, Liu L, Mo BX, Xing YZ, Yu Y, Chen XM. (2022) NAD+-capped RNAs are widespread in rice (Oryza sativa) and spatiotemporally modulated during development. Sci. China-Life Sci., 65: 2121-2124.
Fan LS, Zhang C, Zhang Y, Stewart E, Jez J, Nakajima K, Chen XM. (2022) Microtubules promote the non-cell autonomous action of microRNAs by inhibiting their cytoplasmic loading onto ARGONAUTE1 in Arabidopsis. Dev. Cell., 57: 995-1008.
Liang C, Cai Q, Li SF, You CJ, Xu C, Gao L, Cao DC, Yu Y, Mo BX, Chen XM. (2022) RBV, an evolutionarily conserved WD40 repeat protein, promotes microRNA biogenesis and loading into ARGONAUTE1 in Arabidopsis. Nat. Comm., 13: 1217.
Chen XM, Rechavi O. (2021) Plant and animal small RNA communications between cells and organisms. Nat. Rev. Mol. Cell Biol., 23: 85-203.
Hu H, Flynn N, Chen XM. (2021) Discovery, processing, and potential role of noncanonical caps in RNA. Epitranscriptomics, 12: 435-469.
Hu H, Flynn N, Zhang HL, You CJ, Hang RL, Wang XF, Zhong H, Chan ZL, Xia YJ, Chen XM. (2021) SPAAC-NAD-seq, a sensitive and accurate method to profile NAD+-capped transcripts. PNAS, 118: e2025595118.
Zhang HL, Zhong H, Wang XF, Zhang SD, Shao XJ,Hu H, Yu ZL, Cai ZW, Chen XM, Xia YJ. (2021) Use of NAD tagSeq II to identify growth-phase dependent alterations in E. coli RNA NAD+-capping. PNAS, 118: e2026183118.
Zhang BL, Chen XM. (2021) Secrets of theMIR172family in plant development and flowering unveiled. Plos Biol., 19: e3001099.
Yang XY, You CJ, Wang XF, Gao L, Mo BX, Liu L, Chen XM. (2021) Widespread occurrence of microRNA-mediated target cleavage on membrane-bound polysomes. Genome Biol., 22: 15.
Zhang C, Fan LS, Le BH, Ye PY, Mo BX, Chen XM. (2020) Regulation ofARGONAUTE10expression enables temporal and spatial precision in axillary meristem initiation in Arabidopsis. Dev. Cell, 55: 603-616.
Su ZX, Wang NN, Hou ZM, Li BY, Li DN, Liu YH, Cai HY, Qin Y, Chen XM. (2020) Regulation of female germline specification via small RNA mobility in Arabidopsis. Plant Cell, 32: 2842-2854.
Zhang BL, You CJ, Zhang Y, Zeng LP, Hu J, Zhao ML, Chen XM. (2020) Linking key steps of microRNA biogenesis by TREX-2 and the nuclear pore complex in Arabidopsis. Nat. Plants, 6: 957-969.
Yu Yu, Xufeng Wang, Hao Hu, Jie Huang, Shangze Li, Huichao Liu, Qianyu Wang, Shuang Li, Yiwen Guo, Runlai Hang, Runyu Wang, Li Wang, Aolin Xie, Qiyue Zhang, Lingxiang Chen, Yiran Wang, Huiyuan Lin, Nan Li, Ha n Wang, Xinyue Yu